Getting Stiff: A Revisionist Approach to Stretching & Flexibility - Part 1
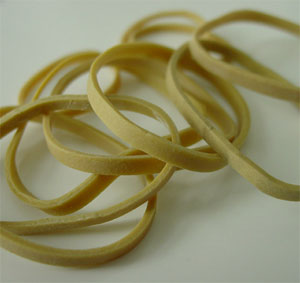
The reigning notion of flexibility and stretching is that its relationships to both athletic performance and injury protection are positive and linear; that is, as stretching and flexibility increase, so do athletic performance and injury protection. With this in mind, advice from nearly everyone for nearly everyone is to stretch as much as possible.
The actual research and experience of coaches and athletes, however, have failed to convincingly demonstrate this or any other relationship. The research hasn't done much of anything beyond making the subject even more confusing by producing a continual stream of contradictory conclusions, and it's easy to find coaches and athletes willing to supply emphatic anecdotal support for any conclusion you'd like.
Research in the arenas of fitness and nutrition are inherently difficult simply because of the enormous number of variables involved and the impossibility of true control subjects. Studies of stretching and flexibility are no exception. In fact, they offer a number of unique problems in addition to those more universal. Studies of stretching's effects on injury prevention and athletic performance have extremely limited application and are not exceptionally reliable. Anecdotal evidence simply contributes another layer of corroboration and contradiction.
Ultimately both coaches and athletes need a reliable flexibility and stretching prescription regardless of how difficult its development may be. In this issue, we'll discuss the research, some biomechanics and other fundamentals related to stretching and flexibility to build a solid foundation on which we'll develop the appropriate prescription in the next issue.
Definitions
The first step through the sludge of stretching and flexibility is to clearly understand the terminology involved. Often the definitions of flexibility and related terms are remarkably flexible. Unfortunately, the degree of flexibility of a term's meaning is proportional to the term's usefulness: The less precise the definition, the less useful the term. To avoid confusion, we're going to clearly define a few terms for the purposes of this discussion.
Flexibility: The degree to which a muscle can be extended beyond its resting length, which will have a positive relationship with the range of motion of the associated joint(s).
Range of Motion: The degree to which the body can move about a given joint.
Stretching: The set of several methods of increasing flexibility.
Hypermobility: More often called Joint Hypermobility. Decreased joint integrity and excessive possible joint movement as a result of congenitally defective connective tissue development.
Acquired Hypermobility: Non-congenital joint hypermobility arising from injury or joint abuse.
Minimal Flexibility: The minimal degree of flexibility required to properly achieve and maintain specified positions and ranges of motion.
Optimal Flexibility: The degree of flexibility for a particular pursuit that allows the greatest performance and provides the greatest injury protection possible.
Hypoflexibility: Flexibility to a degree short of minimal flexibility.
Hyperflexibility: Flexibility to a degree beyond optimal flexibility.
The terms stretching and flexibility are often used interchangeably in reference to research. For reasons that will become clearer as we progress, this is a serious mistake: they are not the same thing. The terms hypermobility and hyperflexibility are also often used synonymously, but they are not the same condition, and the distinction between the two is only somewhat less crucial than between stretching and flexibility. Hyperflexibility refers exclusively to muscle characteristics: more specifically, a muscle's capacity to be lengthened beyond what has been determined to be its ideal maximum length. Hypermobility, whether congenital or acquired, refers to the joint structure and the excessive possible motion and diminished integrity thereof. The reasons for my insistence on clearly distinguishing the two will become more apparent later.
The Research
Relatively little research on the effects of stretching and flexibility on injury prevention and athletic performance exists. That which does exist provides conclusive evidence of virtually nothing. Particularly in the realm of injury prevention, the research demonstrates little other than that it's nearly impossible to conduct useful, reliable and broadly applicable research of this nature.
Studies can be categorized according to their focus on the contributions of either stretching or flexibility to either injury prevention or athletic performance. It's important here to reiterate the distinction between stretching and flexibility: stretching is an activity typically employed as a means to increase flexibility; flexibility is the actual capacity for a muscle to extend beyond its resting length, which is associated with the range of motion of the body about the corresponding joint or joints. They are absolutely not the same thing.
The structure of studies and the wording of their conclusions are critical. For example, we cannot misinterpret a study that compared the injury rates of two groups, one who stretched and one who didn't, that concluded that stretching was associated with decreased rate of injury to mean that increased flexibility reduces the risk of injury. Nor can we misinterpret a study concluding that increased flexibility improved a certain aspect of performance in a certain group of athletes to mean that stretching improves athletic performance. And we cannot misinterpret a study that determined stretching was associated with any effects on injury prevention or performance to mean that all types of stretching will produce the same effects. We must be extremely careful to use the data and conclusions of studies for exactly what they are and nothing more, provided of course that we deem the studies reliable at all.
Let's consider a varied sample of the available research.
A two-year cohort study of over 100 high-level soccer players, Gaelic football players and hurlers determined that "flexibility scores were not found to be significant predictors of injury." An RCT study with about 1500 army recruits for 12 weeks had the test group perform pre-exercise static stretching, while the control performed no stretching. Both groups performed a warm-up prior to stretching and/or exercise. The researchers concluded that "a typical muscle stretching protocol performed during pre-exercise warm-ups does not produce clinically meaningful reductions in risk of exercise-related injury in army recruits."
A study similar to the last of about 300 military trainees, in which the test group performed three hamstring stretching sessions in addition to the normal PT program through which both they and the control group went, concluded that "the number of lower extremity overuse injuries was significantly lower in infantry basic trainees with increased hamstring flexibility." A cohort study of Belgian soccer players during a single season determined that "players with an increased tightness of the hamstring or quadriceps muscles have a statistically higher risk for a subsequent musculoskeletal lesion" A study of a Division III collegiate football team comparing the injury rates of players during two successive seasons, during the second of which the players added static stretching prior to training, found that there was an "association between the incorporation of a static stretching program and a decreased incidence of musculotendinous strains."
An epidemiological study of military personnel data cited both low and high degrees of flexibility as factors for injury risk.
It has been demonstrated that passive stretching may temporarily disrupt nerve function, resulting in diminished force production capacity and delayed reaction to proprioceptive input. This means the possibility of slightly less strength and power and greater injury risk if passive stretching is performed prior to activity. A study using college sprinters similarly found that sprinting speeds following passive stretching were reduced. Another study found that vertical jump height diminished immediately following passive stretching. Research regarding flexibility and running economy—cardiovascular efficiency—hasn't generated consistent conclusions. A study of sixteen powerlifters concluded that increased flexibility improved bench press performance over time.
The Bottom Line
These are just a few of maybe a hundred studies. While not an exhaustive sampling, it is accurately representative of the existing research and should help make clear its overall inconclusiveness.
Studies regarding flexibility and injury risk have almost invariably attempted to demonstrate infinitely linear relationships. In other words, they have sought to show that increasing flexibility or the practice of stretching either increases, decreases or doesn't affect the risk of injury, and that this relationship is the same regardless of the degree of flexibility. But what if the relationship—if there even is a relationship—isn't linear? What if there are static points along the plot of increasing flexibility at which the relative relationship suddenly shifts in terms of its rate of change or even direction?
There is also a great deal of variation among existing studies in terms of purpose, subjects of measurement and evaluation, the methods of measurement and evaluation, the subjects, the types of activities, the frequency and intensity of the activities, the duration of the study, and, possibly most important, there is enormous variation in the actual stretching protocols prescribed to the test subjects or studied retrospectively. Some studies focus merely on the presence or absence of any type of stretching in an athlete's training, some focus on the incorporation of a defined stretching protocol—which may or may not align with what we deem appropriate—and others focus exclusively on the degree of flexibility possessed by the athletes regardless of the methods employed to obtain it. These are all very different things and the associated data communicate very different messages.
On average, studies undertaken in an effort to determine the effects of flexibility and stretching on athletic performance are more useful than their injury counterpart studies: these studies more often focus on the given intervention's effects on specific physiological characteristics, such as those cited above regarding the effects of pre-activity static stretching on strength and power production.
Ultimately, there has been one unequivocal conclusion generated by the existing research: Pre-activity static stretching has the potential to negatively affect factors of athletic performance such as strength and proprioception. This conclusion is transferable to the realm of injury prevention because a reduction of normal strength and proprioception obviously both subject an athlete to a greater risk of injury.
In short, it can be asserted that static stretching generally has no place in pre-training activity, but all other relationships remain less certain according to research results.
Some Biomechanics
Let's briefly indulge in some biomechanics to illustrate some of the most important aspects of optimal flexibility.
The spine is a vertical stack of bone segments that articulate on cartilaginous disks. In its normal, stable position, it curves through lordosis in the lumbar region and kyphosis in the thoracic region. In this naturally curved position, compressive forces are balanced over the surfaces of the vertebrae and intervertebral disks. Maintenance of these curves provides the greatest possible structural integrity, while shifting of them transfers increased pressure either ventrally or dorsally to smaller areas of the vertebrae and disks, reducing structural integrity and increasing the risk of damage. Additionally, the spinal erectors' mechanical disadvantage is exaggerated when the lower back loses its lordotic curve—back strength is greatest with the spine in its normal curvature.
In its natural posture, the spine is curved through kyphosis in the thoracic region and lordosis in the lumbar region. Maintenance of these natural curves provides the greatest possible structural integrity and mechanical advantage, while shifting of them transfers increased pressure either ventrally or dorsally to smaller areas of the vertebrae and disks, reducing structure integrity, exaggerating mechanical disadvantage, and increasing the risk of damage.
The vast majority of back injuries involve the vertebrae L4 to S1—the point at which the spine and pelvis join. The primary reason is pervasive hip extensor and flexor inflexibility, which prevents proper spinal curvature in anything but an upright position and results in hypermobility of the lumbar spine, which must compensate for the lack of pelvic rotation during body flexion.
Flexion and extension can occur at both the hips and the lower spine. Differentiating between the two movements is remarkably difficult for many people. When I ask my clients to point to their hips, their fingers nearly invariably land on the iliac crests of their pelvises—our sense of anatomy has been distorted by idiomatic English. The hip is the joint of the femur and the pelvis, well below the bony ridges we mistakenly refer to as our hips. Were I to draw a line around the circumference of their bodies at this point, it would be right around the L4 – S1 vertebrae—the joint of the spine to the pelvis. (Figure 3)
Public education regarding lifting technique centers on the trite, oversimplified instructions to lift with the legs. But employing the legs in a lifting motion does not necessarily place the back in a biomechanically sound position as the technique ostensibly intends. The ineffectiveness of this advice is related to the misunderstanding of the involved joints as described above.
The lack of distinction between the back and hips becomes problematic during any movement involving bending at the waist ("waist" for our purposes referring to the area comprised of the lumbar spine and hip joints). When the torso changes position relative to the legs, as it does when we squat or lean over to pick something up, little or no control is exercised by the average person regarding at which joint this flexion occurs. This results in the bulk of the work being performed by the joint offering the least resistance—in most cases, the lumbar spine.
This is the primary source of the acquired lumbar hypermobility mentioned earlier. If the pelvis is not allowed to rotate adequately by tight hip extensors, the lower back must hyperflex, reversing the natural lordotic arch. It should be easy to see how the frequent repetition of this movement over many years can result in serious laxity in the lumbar spine, all while enabling the hamstrings—unusually resistant to stretching as it is—to grow even tighter.
No matter how strong your spinal erectors are, their activation across a hypermobile spine simply cannot overcome the far greater strength and relative mechanical advantage of the hamstrings. This means that strengthening of the spinal erectors, while absolutely integral to resolving this problem, cannot be considered a solitary solution—it must be accompanied by increased hamstring flexibility. Nor is the problem resolvable simply by "fighting" the stubbornly static pelvis position while performing the motions during which the problem arises. Direct hamstring stretching is an absolute requirement.
The best illustration of this—partly because it's the most common situation in which this "fighting" is posited as an independent solution—is the squat. As the depth of the hips increases during a squat, the angle of the back relative to the upper legs decreases, demanding lengthening of the hamstrings. If the hamstrings are not adequately flexible, they will pull the pelvis with them, leaving the lumbar spine to absorb the remaining necessary flexion. No amount of fighting with the strength of the mechanically disadvantaged spinal erectors over a hypermobile spine will defeat the strength and relative mechanical advantage of short hamstrings.
The idea that improper squat positioning due to inflexibility can be fixed by nothing more than doing squats to only the greatest depth at which lordosis can be maintained and then attempting to incrementally increase that depth over time while maintaining lordosis is not sound. While squatting will increase strength in both the spinal erectors and hamstrings, this ratio of strength and mechanical advantage will remain constant, resulting in no net improvement of the spinal erectors' ability to fight the hamstrings. Compounding the problem is the limited range of motion, which will encourage further shortening of the hamstrings.
And now another joint gets involved: the knee. When performing a proper, full range of motion squat, the knees are not subjected to any excessive degree or unusual type of force. The squat is an entirely natural movement and position for the human body, and, performed properly, will fortify the structures and abilities of the body, not harm them. That doesn't mean, however, that all variations of the squat are equally beneficial or without risk. If a squat is stopped short of full depth, undue stress is placed on the knees.
In a properly-positioned full-depth squat (hips below the knees), tension in the hamstrings balances the forces on the knee joint by opposing the force of the quadriceps. If the depth of the squat is not sufficient to generate this hamstring tension, the tension of the quadriceps on the knee is disproportionately great, resulting in potentially damaging shear force.
In short, as a method of attaining adequate flexibility for proper movement and position in the squat, the protocol of squatting only to the depth at which lordosis can be maintained and attempting to incrementally increase that depth over time is ineffective at best and potentially harmful at worst. The protocol must include additional stretching.
While hypoflexibility can create serious problems as described above, hyperflexibility may not necessarily be a substantially better condition.
The most obvious potential problem is related to the above discussion of the undue stress to the knees in the partial squat. As described there, hamstring tension at the bottom of the squat provides knee stability by balancing the forces on the joint. With hypoflexible hamstrings, if a full-depth squat is performed, this hamstring tension is not developed because of pelvic rotation shortening the distance between the muscles' origins and insertions, whereas if a partial depth squat is performed to maintain lordosis, the hamstrings are also not lengthened sufficiently to generate the necessary tension.
On the other hand, hyperflexible hamstrings may also fail to develop adequate tension on the tibia in this bottom position, thereby contributing to similar shear force on the knee. However, while the potential for this shear force is similar with hypoflexible and hyperflexible hamstrings, hyperflexibility is far less of a concern: with conscious effort, the hamstrings can be activated sufficiently throughout the squat to create the necessary tension, and, in fact, this activation of the hamstrings is requisite to a proper squat.
Some Finer Points
My explanation to many of my clients that they have hypoflexible hamstrings, hyperflexible spinal erectors, and hypermobile lumbar spines is often countered by complaints of lower back tightness, and insistence that their spinal erectors must therefore be stretched and their abdominals strengthened. But the naming of tight spinal erectors as the cause of lumber spine tightness is commonly erroneous. More often than not, the actual source of their discomfort is a combination of tight psoas, hamstrings or a combination of the two.
The psoas is a hip flexor that inserts with the iliacus on the femur, originating on the inferior thoracic and lumbar vertebrae. Tension in the muscle pulls these vertebrae toward the leg, resulting in an exaggeration of the lumbar curve called hyperlordosis—or Hairdresser Back—and the associated feeling of a tight lower back.
The psoas originates along the inferior thoracic and lumbar vertebrae and insert on the femur to serve as a hip flexor. Tight psoas exaggerates the lordotic arch, creating compression in the lower back. This is often mistaken for tight spinal erectors.
Responding as is typical to this condition by stretching the lower back and performing more abdominal—or worse, hip flexion—work compounds the problem by reducing the stabilizing pull of the spinal erectors, strengthening the psoas, and increasing the mobility of the likely already hypermobile lumbar spine, exacerbating existing hyperlordosis and the feeling of back compression.
If the hamstrings are also tight, the summation of their tension on the pelvis and the tension of the psoas on the lumbar vertebrae further exaggerates the compression of the lumbar spine. In this case, posture may actually appear correct in terms of pelvic rotation and spinal curvature because of the balance of anterior and posterior tension. But while the position is normal, the amount of compressive force on the spine is not.
It's critical to be aware of what is and isn't actually tight, and to stretch and strengthen accordingly. Assessments and programming will be discussed and demonstrated in the next issue.
Flexibility and Stretching: The Revised Version
It's my contention that the relationship of flexibility to both injury and performance describes a modified bell curve; that is, both hypoflexibility and hyperflexibility increase the risk of injury and may limit performance. However, in close proximity to the apex representing optimal flexibility—the degree of flexibility associated with the least risk of injury and the greatest performance—hypoflexibility is associated with greater injury risk and greater performance inhibition than is hyperflexibility.
The relationship of injury protection and performance to flexibility describes a modified bell curve. The apex represents optimal flexibility—the degree of flexibility that allows the greatest performance and provides the greatest protection from injury. Note that the curve is steeper on the hypoflexibility side of the apex—in proximity to optimal flexibility, a given degree of hypoflexibility impedes performance and increases the risk of injury to a greater extent than that same degree of hyperflexibility.
Evidence that has shown stretching to be associated with increased injury rates can be explained by improper flexibility programming and implementation. In short, the increased rates of injuries were due to joint instability or muscle damage caused by improper stretching or from the negative effects of pre-activity static stretching—not a state of hyperflexibility. The vast majority of studies testing whether or not stretching reduces the risk of injury have involved pre-activity static stretching, which, as mentioned earlier, increases injury risk regardless of a subject's flexibility. Additionally, stretching is an activity that very few people do correctly, exposing their connective tissue to potentially damaging stress, as we'll discuss in greater detail in the next issue.
Another part of my resistance to believing hyperflexibility to be the cause of increased injury rates in these studies is the simple fact that I have yet to meet a single human being, athlete or otherwise, who could be considered hyperflexible. I've seen photographs of Thomas Kurz doing the splits in between two folding chairs, but that position is anything but common. In my experience, the vast majority of people—including long-time, competitive athletes—are actually surprisingly inflexible, and, more importantly, possess unbalanced degrees of flexibility among joints. They are unable to properly achieve positions and ranges of motion demanded by their sports, and are therefore accurately described as hypoflexible, not hyperflexible as careless interpretations of stretching studies may suggest.
Some research, like the powerlifter study cited previously, has demonstrated improved performance over time with increased flexibility of the athletes. But these results are very specific to conditions in the study: they don't demonstrate a reliable linear relationship between flexibility and strength, for example. More likely, the athletes in question were hypoflexible to the point of impeded performance, and the flexibility training they performed as test subjects simply reduced that impediment. There is no evidence that further increasing their flexibility would have continued improving their performance.
It's important to understand the difference between reducing or eliminating a performance-limiting factor and actually improving performance. It's also important to visualize this trend on the bell curve described previously and note that past the apex, the increasing flexibility that initially improved performance may begin to harm it instead. In terms of athletic performance, optimal flexibility will not improve in any dramatic manner strength, power, speed or any other physiological capacity. It will simple eliminate hypoflexibility-related performance limitations. If those limitations in a particular athlete are extraordinarily great, however, the improvement in performance gained from increased flexibility may be dramatic.
Requirements
One of the key parameters necessary to developing a proper stretching prescription is an athlete's flexibility demands. There are two categories of flexibility requirements—universal and sport-specific. That is, there is a defined set of positions and ranges of motion that are requisite to functional, healthy human life, and these obviously apply to everyone of human genetic construction. And then there is a set of positions and ranges of motions that are defined by the demands of your chosen athletic pursuits. The degree to which your sport-specific requirements differ from the universal requirements is dependent on the unique demands of your sport.
All athletic activity involves movement and the attainment and sometimes maintenance of certain positions (e.g. static holds in gymnastics). Some sports demand those things in the presence of significant loading or forces far exceeding bodyweight. Sport-specific positions may be functional, reflective to varying degrees of some of our universal positions and movements, while others may be wholly non-functional in this sense.
For example, a flare in gymnastics is a movement demanding of a high degree of flexibility, but it is not functional in the fundamental sense of the word—it's not a movement the human body would ever need to perform in the course of practical existence. A squat, on the other hand, is found in numerous variations in many sports, but also epitomizes functionality.
The reason this idea is important is that when determining your flexibility requirements, you need to first recognize and understand the demands of your existence and their demands of flexibility. In most cases, your sport-specific flexibility requirements will involve a greater degree of flexibility than the universal requirements, but this is not a rule without exception. If you do nothing more than run, for example, you have relatively little demand for athletic flexibility; your sport requires a very limited range of motion of all joints. On the other end of the spectrum are sports like gymnastics and Olympic weightlifting, which demand movement and the control of great resistance through ranges of motion beyond those found in the universal requirements.
The manner in which optimal flexibility improves athletic performance also varies greatly depending on your sport, but more importantly, on its relation to your previous flexibility status. As I suggested earlier in reference to the powerlifters whose bench press performance improved with the incorporation of stretching into their training, optimal flexibility is not technically a performance enhancer, but an impediment reducer. This is important to keep in mind when considering the flexibility-injury-performance curve: increasing flexibility will increase performance and decrease risk of injury if you are presently hypoflexible. But that trend will not continue indefinitely. Beyond the apex of the curve representing optimal flexibility, the trend prior will invert, and it's likely that increasing flexibility will increase the risk of injury and decrease performance.
My focus within the context of injury protection is connective tissue injury. That said, I don't disregard strains entirely. A severe enough strain can cause long term or permanent deformity and compromise function. But I would argue that strains of that severity are rarer and more difficult to incur than connective tissue injuries. My primary concern is actually not severe, acute injuries, but long term or even permanent damage to ligaments and joint structures. More specifically, my focus within the context of joint structure injury is cumulative connective tissue damage, which, in my opinion, is far more insidious than even fairly severe acute injury.
For example, if you rupture your ACL, you will most likely notice. The condition is relatively easy to diagnose reliably, and surgery to repair the rupture and subsequent rehabilitation seem to fairly successful. On the other hand (or leg), consider gradual loosening of knee ligaments due to some unspecified activity repeated regularly over a long period of time. No acute injury and its associated sudden pain, noise or unnatural joint movement exists to alert you of a problem that you can then address; the minutely incremental lengthening of the ligaments is completely unnoticeable day to day, and it therefore continues unnoticed until an acute injury, facilitated by this condition, occurs and suddenly draws your attention to your unusual joint laxity.
By this point, the ligaments are probably going to stay stretched. Reattaching a ligament is one thing—making a ligament shorten is another. Thermal capsulorrhaphy—surgical application of heat to the joint capsule to cause microscopic restructuring and subsequent tightening of the joint might work. Of course it also poses the risks of motor nerve damage, over-tightening, and burns to a degree beyond reconstruction. The only practical solution to joint laxity is preventing it.
The Y-Word
I would be derelict in my duties if I failed to discuss at least briefly the Y-word. Yoga has somehow developed a reputation of being a legitimate method of developing flexibility, at least among people with no exposure to real stretching. The truth is that it's surprisingly ineffective. More importantly, its positions frequently violate what I consider the single most crucial rule of flexibility training: stretching shall never place undue stress on connective tissue or joints. I won't argue that yoga may be a wonderful way to relax and relieve stress. I have no intention of denying anyone stress reduction; as the Cortisol Kid, I encourage it emphatically. But there are many methods of stress reduction that do not concurrently damage the body. I assure you that experiencing a joint injury precipitated by lax connective tissue will not reduce your stress levels.
The Bottom Line
In short, a flexibility prescription is concerned with two areas: injury prevention and performance impediment elimination. In terms of injury prevention, the goal is to reduce the likelihood of musculotendinous, ligamental or other injuries, with its primary focus being the reduction of potentially damaging stress to connective tissue and the maintenance of joint integrity. In terms of performance, the goal is to eliminate any impediments arising from either hypo- or hyperflexibility.
In order to achieve these goals, the prescription must be predicated on determined flexibility requirements, of which there are two types: universal and specific. Universal requirements are those that apply to everyone; specific requirements are unique to each sport or activity and must be determined individually for each athlete. The protocol must employ proper stretching methods that achieve the desired goals but do not at all threaten joint integrity or performance.
Until Next Time
That's it for this installment. In the next issue we'll finish the process with a revised flexibility training prescription based on the information presented in this article, including flexibility assessments and how and when to perform what stretches. Until then, lay off the pre-activity static stretching and drop out of your yoga class.
The actual research and experience of coaches and athletes, however, have failed to convincingly demonstrate this or any other relationship. The research hasn't done much of anything beyond making the subject even more confusing by producing a continual stream of contradictory conclusions, and it's easy to find coaches and athletes willing to supply emphatic anecdotal support for any conclusion you'd like.
Research in the arenas of fitness and nutrition are inherently difficult simply because of the enormous number of variables involved and the impossibility of true control subjects. Studies of stretching and flexibility are no exception. In fact, they offer a number of unique problems in addition to those more universal. Studies of stretching's effects on injury prevention and athletic performance have extremely limited application and are not exceptionally reliable. Anecdotal evidence simply contributes another layer of corroboration and contradiction.
Ultimately both coaches and athletes need a reliable flexibility and stretching prescription regardless of how difficult its development may be. In this issue, we'll discuss the research, some biomechanics and other fundamentals related to stretching and flexibility to build a solid foundation on which we'll develop the appropriate prescription in the next issue.
Definitions
The first step through the sludge of stretching and flexibility is to clearly understand the terminology involved. Often the definitions of flexibility and related terms are remarkably flexible. Unfortunately, the degree of flexibility of a term's meaning is proportional to the term's usefulness: The less precise the definition, the less useful the term. To avoid confusion, we're going to clearly define a few terms for the purposes of this discussion.
Flexibility: The degree to which a muscle can be extended beyond its resting length, which will have a positive relationship with the range of motion of the associated joint(s).
Range of Motion: The degree to which the body can move about a given joint.
Stretching: The set of several methods of increasing flexibility.
Hypermobility: More often called Joint Hypermobility. Decreased joint integrity and excessive possible joint movement as a result of congenitally defective connective tissue development.
Acquired Hypermobility: Non-congenital joint hypermobility arising from injury or joint abuse.
Minimal Flexibility: The minimal degree of flexibility required to properly achieve and maintain specified positions and ranges of motion.
Optimal Flexibility: The degree of flexibility for a particular pursuit that allows the greatest performance and provides the greatest injury protection possible.
Hypoflexibility: Flexibility to a degree short of minimal flexibility.
Hyperflexibility: Flexibility to a degree beyond optimal flexibility.
The terms stretching and flexibility are often used interchangeably in reference to research. For reasons that will become clearer as we progress, this is a serious mistake: they are not the same thing. The terms hypermobility and hyperflexibility are also often used synonymously, but they are not the same condition, and the distinction between the two is only somewhat less crucial than between stretching and flexibility. Hyperflexibility refers exclusively to muscle characteristics: more specifically, a muscle's capacity to be lengthened beyond what has been determined to be its ideal maximum length. Hypermobility, whether congenital or acquired, refers to the joint structure and the excessive possible motion and diminished integrity thereof. The reasons for my insistence on clearly distinguishing the two will become more apparent later.
The Research
Relatively little research on the effects of stretching and flexibility on injury prevention and athletic performance exists. That which does exist provides conclusive evidence of virtually nothing. Particularly in the realm of injury prevention, the research demonstrates little other than that it's nearly impossible to conduct useful, reliable and broadly applicable research of this nature.
Studies can be categorized according to their focus on the contributions of either stretching or flexibility to either injury prevention or athletic performance. It's important here to reiterate the distinction between stretching and flexibility: stretching is an activity typically employed as a means to increase flexibility; flexibility is the actual capacity for a muscle to extend beyond its resting length, which is associated with the range of motion of the body about the corresponding joint or joints. They are absolutely not the same thing.
The structure of studies and the wording of their conclusions are critical. For example, we cannot misinterpret a study that compared the injury rates of two groups, one who stretched and one who didn't, that concluded that stretching was associated with decreased rate of injury to mean that increased flexibility reduces the risk of injury. Nor can we misinterpret a study concluding that increased flexibility improved a certain aspect of performance in a certain group of athletes to mean that stretching improves athletic performance. And we cannot misinterpret a study that determined stretching was associated with any effects on injury prevention or performance to mean that all types of stretching will produce the same effects. We must be extremely careful to use the data and conclusions of studies for exactly what they are and nothing more, provided of course that we deem the studies reliable at all.
Let's consider a varied sample of the available research.
A two-year cohort study of over 100 high-level soccer players, Gaelic football players and hurlers determined that "flexibility scores were not found to be significant predictors of injury." An RCT study with about 1500 army recruits for 12 weeks had the test group perform pre-exercise static stretching, while the control performed no stretching. Both groups performed a warm-up prior to stretching and/or exercise. The researchers concluded that "a typical muscle stretching protocol performed during pre-exercise warm-ups does not produce clinically meaningful reductions in risk of exercise-related injury in army recruits."
A study similar to the last of about 300 military trainees, in which the test group performed three hamstring stretching sessions in addition to the normal PT program through which both they and the control group went, concluded that "the number of lower extremity overuse injuries was significantly lower in infantry basic trainees with increased hamstring flexibility." A cohort study of Belgian soccer players during a single season determined that "players with an increased tightness of the hamstring or quadriceps muscles have a statistically higher risk for a subsequent musculoskeletal lesion" A study of a Division III collegiate football team comparing the injury rates of players during two successive seasons, during the second of which the players added static stretching prior to training, found that there was an "association between the incorporation of a static stretching program and a decreased incidence of musculotendinous strains."
An epidemiological study of military personnel data cited both low and high degrees of flexibility as factors for injury risk.
It has been demonstrated that passive stretching may temporarily disrupt nerve function, resulting in diminished force production capacity and delayed reaction to proprioceptive input. This means the possibility of slightly less strength and power and greater injury risk if passive stretching is performed prior to activity. A study using college sprinters similarly found that sprinting speeds following passive stretching were reduced. Another study found that vertical jump height diminished immediately following passive stretching. Research regarding flexibility and running economy—cardiovascular efficiency—hasn't generated consistent conclusions. A study of sixteen powerlifters concluded that increased flexibility improved bench press performance over time.
The Bottom Line
These are just a few of maybe a hundred studies. While not an exhaustive sampling, it is accurately representative of the existing research and should help make clear its overall inconclusiveness.
Studies regarding flexibility and injury risk have almost invariably attempted to demonstrate infinitely linear relationships. In other words, they have sought to show that increasing flexibility or the practice of stretching either increases, decreases or doesn't affect the risk of injury, and that this relationship is the same regardless of the degree of flexibility. But what if the relationship—if there even is a relationship—isn't linear? What if there are static points along the plot of increasing flexibility at which the relative relationship suddenly shifts in terms of its rate of change or even direction?
There is also a great deal of variation among existing studies in terms of purpose, subjects of measurement and evaluation, the methods of measurement and evaluation, the subjects, the types of activities, the frequency and intensity of the activities, the duration of the study, and, possibly most important, there is enormous variation in the actual stretching protocols prescribed to the test subjects or studied retrospectively. Some studies focus merely on the presence or absence of any type of stretching in an athlete's training, some focus on the incorporation of a defined stretching protocol—which may or may not align with what we deem appropriate—and others focus exclusively on the degree of flexibility possessed by the athletes regardless of the methods employed to obtain it. These are all very different things and the associated data communicate very different messages.
On average, studies undertaken in an effort to determine the effects of flexibility and stretching on athletic performance are more useful than their injury counterpart studies: these studies more often focus on the given intervention's effects on specific physiological characteristics, such as those cited above regarding the effects of pre-activity static stretching on strength and power production.
Ultimately, there has been one unequivocal conclusion generated by the existing research: Pre-activity static stretching has the potential to negatively affect factors of athletic performance such as strength and proprioception. This conclusion is transferable to the realm of injury prevention because a reduction of normal strength and proprioception obviously both subject an athlete to a greater risk of injury.
In short, it can be asserted that static stretching generally has no place in pre-training activity, but all other relationships remain less certain according to research results.
Some Biomechanics
Let's briefly indulge in some biomechanics to illustrate some of the most important aspects of optimal flexibility.
The spine is a vertical stack of bone segments that articulate on cartilaginous disks. In its normal, stable position, it curves through lordosis in the lumbar region and kyphosis in the thoracic region. In this naturally curved position, compressive forces are balanced over the surfaces of the vertebrae and intervertebral disks. Maintenance of these curves provides the greatest possible structural integrity, while shifting of them transfers increased pressure either ventrally or dorsally to smaller areas of the vertebrae and disks, reducing structural integrity and increasing the risk of damage. Additionally, the spinal erectors' mechanical disadvantage is exaggerated when the lower back loses its lordotic curve—back strength is greatest with the spine in its normal curvature.
In its natural posture, the spine is curved through kyphosis in the thoracic region and lordosis in the lumbar region. Maintenance of these natural curves provides the greatest possible structural integrity and mechanical advantage, while shifting of them transfers increased pressure either ventrally or dorsally to smaller areas of the vertebrae and disks, reducing structure integrity, exaggerating mechanical disadvantage, and increasing the risk of damage.
The vast majority of back injuries involve the vertebrae L4 to S1—the point at which the spine and pelvis join. The primary reason is pervasive hip extensor and flexor inflexibility, which prevents proper spinal curvature in anything but an upright position and results in hypermobility of the lumbar spine, which must compensate for the lack of pelvic rotation during body flexion.
Flexion and extension can occur at both the hips and the lower spine. Differentiating between the two movements is remarkably difficult for many people. When I ask my clients to point to their hips, their fingers nearly invariably land on the iliac crests of their pelvises—our sense of anatomy has been distorted by idiomatic English. The hip is the joint of the femur and the pelvis, well below the bony ridges we mistakenly refer to as our hips. Were I to draw a line around the circumference of their bodies at this point, it would be right around the L4 – S1 vertebrae—the joint of the spine to the pelvis. (Figure 3)
Public education regarding lifting technique centers on the trite, oversimplified instructions to lift with the legs. But employing the legs in a lifting motion does not necessarily place the back in a biomechanically sound position as the technique ostensibly intends. The ineffectiveness of this advice is related to the misunderstanding of the involved joints as described above.
The lack of distinction between the back and hips becomes problematic during any movement involving bending at the waist ("waist" for our purposes referring to the area comprised of the lumbar spine and hip joints). When the torso changes position relative to the legs, as it does when we squat or lean over to pick something up, little or no control is exercised by the average person regarding at which joint this flexion occurs. This results in the bulk of the work being performed by the joint offering the least resistance—in most cases, the lumbar spine.
This is the primary source of the acquired lumbar hypermobility mentioned earlier. If the pelvis is not allowed to rotate adequately by tight hip extensors, the lower back must hyperflex, reversing the natural lordotic arch. It should be easy to see how the frequent repetition of this movement over many years can result in serious laxity in the lumbar spine, all while enabling the hamstrings—unusually resistant to stretching as it is—to grow even tighter.
No matter how strong your spinal erectors are, their activation across a hypermobile spine simply cannot overcome the far greater strength and relative mechanical advantage of the hamstrings. This means that strengthening of the spinal erectors, while absolutely integral to resolving this problem, cannot be considered a solitary solution—it must be accompanied by increased hamstring flexibility. Nor is the problem resolvable simply by "fighting" the stubbornly static pelvis position while performing the motions during which the problem arises. Direct hamstring stretching is an absolute requirement.
The best illustration of this—partly because it's the most common situation in which this "fighting" is posited as an independent solution—is the squat. As the depth of the hips increases during a squat, the angle of the back relative to the upper legs decreases, demanding lengthening of the hamstrings. If the hamstrings are not adequately flexible, they will pull the pelvis with them, leaving the lumbar spine to absorb the remaining necessary flexion. No amount of fighting with the strength of the mechanically disadvantaged spinal erectors over a hypermobile spine will defeat the strength and relative mechanical advantage of short hamstrings.
The idea that improper squat positioning due to inflexibility can be fixed by nothing more than doing squats to only the greatest depth at which lordosis can be maintained and then attempting to incrementally increase that depth over time while maintaining lordosis is not sound. While squatting will increase strength in both the spinal erectors and hamstrings, this ratio of strength and mechanical advantage will remain constant, resulting in no net improvement of the spinal erectors' ability to fight the hamstrings. Compounding the problem is the limited range of motion, which will encourage further shortening of the hamstrings.
And now another joint gets involved: the knee. When performing a proper, full range of motion squat, the knees are not subjected to any excessive degree or unusual type of force. The squat is an entirely natural movement and position for the human body, and, performed properly, will fortify the structures and abilities of the body, not harm them. That doesn't mean, however, that all variations of the squat are equally beneficial or without risk. If a squat is stopped short of full depth, undue stress is placed on the knees.
In a properly-positioned full-depth squat (hips below the knees), tension in the hamstrings balances the forces on the knee joint by opposing the force of the quadriceps. If the depth of the squat is not sufficient to generate this hamstring tension, the tension of the quadriceps on the knee is disproportionately great, resulting in potentially damaging shear force.
In short, as a method of attaining adequate flexibility for proper movement and position in the squat, the protocol of squatting only to the depth at which lordosis can be maintained and attempting to incrementally increase that depth over time is ineffective at best and potentially harmful at worst. The protocol must include additional stretching.
While hypoflexibility can create serious problems as described above, hyperflexibility may not necessarily be a substantially better condition.
The most obvious potential problem is related to the above discussion of the undue stress to the knees in the partial squat. As described there, hamstring tension at the bottom of the squat provides knee stability by balancing the forces on the joint. With hypoflexible hamstrings, if a full-depth squat is performed, this hamstring tension is not developed because of pelvic rotation shortening the distance between the muscles' origins and insertions, whereas if a partial depth squat is performed to maintain lordosis, the hamstrings are also not lengthened sufficiently to generate the necessary tension.
On the other hand, hyperflexible hamstrings may also fail to develop adequate tension on the tibia in this bottom position, thereby contributing to similar shear force on the knee. However, while the potential for this shear force is similar with hypoflexible and hyperflexible hamstrings, hyperflexibility is far less of a concern: with conscious effort, the hamstrings can be activated sufficiently throughout the squat to create the necessary tension, and, in fact, this activation of the hamstrings is requisite to a proper squat.
Some Finer Points
My explanation to many of my clients that they have hypoflexible hamstrings, hyperflexible spinal erectors, and hypermobile lumbar spines is often countered by complaints of lower back tightness, and insistence that their spinal erectors must therefore be stretched and their abdominals strengthened. But the naming of tight spinal erectors as the cause of lumber spine tightness is commonly erroneous. More often than not, the actual source of their discomfort is a combination of tight psoas, hamstrings or a combination of the two.
The psoas is a hip flexor that inserts with the iliacus on the femur, originating on the inferior thoracic and lumbar vertebrae. Tension in the muscle pulls these vertebrae toward the leg, resulting in an exaggeration of the lumbar curve called hyperlordosis—or Hairdresser Back—and the associated feeling of a tight lower back.
The psoas originates along the inferior thoracic and lumbar vertebrae and insert on the femur to serve as a hip flexor. Tight psoas exaggerates the lordotic arch, creating compression in the lower back. This is often mistaken for tight spinal erectors.
Responding as is typical to this condition by stretching the lower back and performing more abdominal—or worse, hip flexion—work compounds the problem by reducing the stabilizing pull of the spinal erectors, strengthening the psoas, and increasing the mobility of the likely already hypermobile lumbar spine, exacerbating existing hyperlordosis and the feeling of back compression.
If the hamstrings are also tight, the summation of their tension on the pelvis and the tension of the psoas on the lumbar vertebrae further exaggerates the compression of the lumbar spine. In this case, posture may actually appear correct in terms of pelvic rotation and spinal curvature because of the balance of anterior and posterior tension. But while the position is normal, the amount of compressive force on the spine is not.
It's critical to be aware of what is and isn't actually tight, and to stretch and strengthen accordingly. Assessments and programming will be discussed and demonstrated in the next issue.
Flexibility and Stretching: The Revised Version
It's my contention that the relationship of flexibility to both injury and performance describes a modified bell curve; that is, both hypoflexibility and hyperflexibility increase the risk of injury and may limit performance. However, in close proximity to the apex representing optimal flexibility—the degree of flexibility associated with the least risk of injury and the greatest performance—hypoflexibility is associated with greater injury risk and greater performance inhibition than is hyperflexibility.
The relationship of injury protection and performance to flexibility describes a modified bell curve. The apex represents optimal flexibility—the degree of flexibility that allows the greatest performance and provides the greatest protection from injury. Note that the curve is steeper on the hypoflexibility side of the apex—in proximity to optimal flexibility, a given degree of hypoflexibility impedes performance and increases the risk of injury to a greater extent than that same degree of hyperflexibility.
Evidence that has shown stretching to be associated with increased injury rates can be explained by improper flexibility programming and implementation. In short, the increased rates of injuries were due to joint instability or muscle damage caused by improper stretching or from the negative effects of pre-activity static stretching—not a state of hyperflexibility. The vast majority of studies testing whether or not stretching reduces the risk of injury have involved pre-activity static stretching, which, as mentioned earlier, increases injury risk regardless of a subject's flexibility. Additionally, stretching is an activity that very few people do correctly, exposing their connective tissue to potentially damaging stress, as we'll discuss in greater detail in the next issue.
Another part of my resistance to believing hyperflexibility to be the cause of increased injury rates in these studies is the simple fact that I have yet to meet a single human being, athlete or otherwise, who could be considered hyperflexible. I've seen photographs of Thomas Kurz doing the splits in between two folding chairs, but that position is anything but common. In my experience, the vast majority of people—including long-time, competitive athletes—are actually surprisingly inflexible, and, more importantly, possess unbalanced degrees of flexibility among joints. They are unable to properly achieve positions and ranges of motion demanded by their sports, and are therefore accurately described as hypoflexible, not hyperflexible as careless interpretations of stretching studies may suggest.
Some research, like the powerlifter study cited previously, has demonstrated improved performance over time with increased flexibility of the athletes. But these results are very specific to conditions in the study: they don't demonstrate a reliable linear relationship between flexibility and strength, for example. More likely, the athletes in question were hypoflexible to the point of impeded performance, and the flexibility training they performed as test subjects simply reduced that impediment. There is no evidence that further increasing their flexibility would have continued improving their performance.
It's important to understand the difference between reducing or eliminating a performance-limiting factor and actually improving performance. It's also important to visualize this trend on the bell curve described previously and note that past the apex, the increasing flexibility that initially improved performance may begin to harm it instead. In terms of athletic performance, optimal flexibility will not improve in any dramatic manner strength, power, speed or any other physiological capacity. It will simple eliminate hypoflexibility-related performance limitations. If those limitations in a particular athlete are extraordinarily great, however, the improvement in performance gained from increased flexibility may be dramatic.
Requirements
One of the key parameters necessary to developing a proper stretching prescription is an athlete's flexibility demands. There are two categories of flexibility requirements—universal and sport-specific. That is, there is a defined set of positions and ranges of motion that are requisite to functional, healthy human life, and these obviously apply to everyone of human genetic construction. And then there is a set of positions and ranges of motions that are defined by the demands of your chosen athletic pursuits. The degree to which your sport-specific requirements differ from the universal requirements is dependent on the unique demands of your sport.
All athletic activity involves movement and the attainment and sometimes maintenance of certain positions (e.g. static holds in gymnastics). Some sports demand those things in the presence of significant loading or forces far exceeding bodyweight. Sport-specific positions may be functional, reflective to varying degrees of some of our universal positions and movements, while others may be wholly non-functional in this sense.
For example, a flare in gymnastics is a movement demanding of a high degree of flexibility, but it is not functional in the fundamental sense of the word—it's not a movement the human body would ever need to perform in the course of practical existence. A squat, on the other hand, is found in numerous variations in many sports, but also epitomizes functionality.
The reason this idea is important is that when determining your flexibility requirements, you need to first recognize and understand the demands of your existence and their demands of flexibility. In most cases, your sport-specific flexibility requirements will involve a greater degree of flexibility than the universal requirements, but this is not a rule without exception. If you do nothing more than run, for example, you have relatively little demand for athletic flexibility; your sport requires a very limited range of motion of all joints. On the other end of the spectrum are sports like gymnastics and Olympic weightlifting, which demand movement and the control of great resistance through ranges of motion beyond those found in the universal requirements.
The manner in which optimal flexibility improves athletic performance also varies greatly depending on your sport, but more importantly, on its relation to your previous flexibility status. As I suggested earlier in reference to the powerlifters whose bench press performance improved with the incorporation of stretching into their training, optimal flexibility is not technically a performance enhancer, but an impediment reducer. This is important to keep in mind when considering the flexibility-injury-performance curve: increasing flexibility will increase performance and decrease risk of injury if you are presently hypoflexible. But that trend will not continue indefinitely. Beyond the apex of the curve representing optimal flexibility, the trend prior will invert, and it's likely that increasing flexibility will increase the risk of injury and decrease performance.
My focus within the context of injury protection is connective tissue injury. That said, I don't disregard strains entirely. A severe enough strain can cause long term or permanent deformity and compromise function. But I would argue that strains of that severity are rarer and more difficult to incur than connective tissue injuries. My primary concern is actually not severe, acute injuries, but long term or even permanent damage to ligaments and joint structures. More specifically, my focus within the context of joint structure injury is cumulative connective tissue damage, which, in my opinion, is far more insidious than even fairly severe acute injury.
For example, if you rupture your ACL, you will most likely notice. The condition is relatively easy to diagnose reliably, and surgery to repair the rupture and subsequent rehabilitation seem to fairly successful. On the other hand (or leg), consider gradual loosening of knee ligaments due to some unspecified activity repeated regularly over a long period of time. No acute injury and its associated sudden pain, noise or unnatural joint movement exists to alert you of a problem that you can then address; the minutely incremental lengthening of the ligaments is completely unnoticeable day to day, and it therefore continues unnoticed until an acute injury, facilitated by this condition, occurs and suddenly draws your attention to your unusual joint laxity.
By this point, the ligaments are probably going to stay stretched. Reattaching a ligament is one thing—making a ligament shorten is another. Thermal capsulorrhaphy—surgical application of heat to the joint capsule to cause microscopic restructuring and subsequent tightening of the joint might work. Of course it also poses the risks of motor nerve damage, over-tightening, and burns to a degree beyond reconstruction. The only practical solution to joint laxity is preventing it.
The Y-Word
I would be derelict in my duties if I failed to discuss at least briefly the Y-word. Yoga has somehow developed a reputation of being a legitimate method of developing flexibility, at least among people with no exposure to real stretching. The truth is that it's surprisingly ineffective. More importantly, its positions frequently violate what I consider the single most crucial rule of flexibility training: stretching shall never place undue stress on connective tissue or joints. I won't argue that yoga may be a wonderful way to relax and relieve stress. I have no intention of denying anyone stress reduction; as the Cortisol Kid, I encourage it emphatically. But there are many methods of stress reduction that do not concurrently damage the body. I assure you that experiencing a joint injury precipitated by lax connective tissue will not reduce your stress levels.
The Bottom Line
In short, a flexibility prescription is concerned with two areas: injury prevention and performance impediment elimination. In terms of injury prevention, the goal is to reduce the likelihood of musculotendinous, ligamental or other injuries, with its primary focus being the reduction of potentially damaging stress to connective tissue and the maintenance of joint integrity. In terms of performance, the goal is to eliminate any impediments arising from either hypo- or hyperflexibility.
In order to achieve these goals, the prescription must be predicated on determined flexibility requirements, of which there are two types: universal and specific. Universal requirements are those that apply to everyone; specific requirements are unique to each sport or activity and must be determined individually for each athlete. The protocol must employ proper stretching methods that achieve the desired goals but do not at all threaten joint integrity or performance.
Until Next Time
That's it for this installment. In the next issue we'll finish the process with a revised flexibility training prescription based on the information presented in this article, including flexibility assessments and how and when to perform what stretches. Until then, lay off the pre-activity static stretching and drop out of your yoga class.
Greg Everett is the owner of Catalyst Athletics, publisher of The Performance Menu Journal and author of Olympic Weightlifting: A Complete Guide for Athletes & Coaches, Olympic Weightlifting for Sports, and The Portable Greg Everett, and is the writer, director, producer, editor, etc of the independent documentary American Weightlifting. Follow him on Facebook here. |
Search Articles
Article Categories
Sort by Author
Sort by Issue & Date
Article Categories
Sort by Author
Sort by Issue & Date